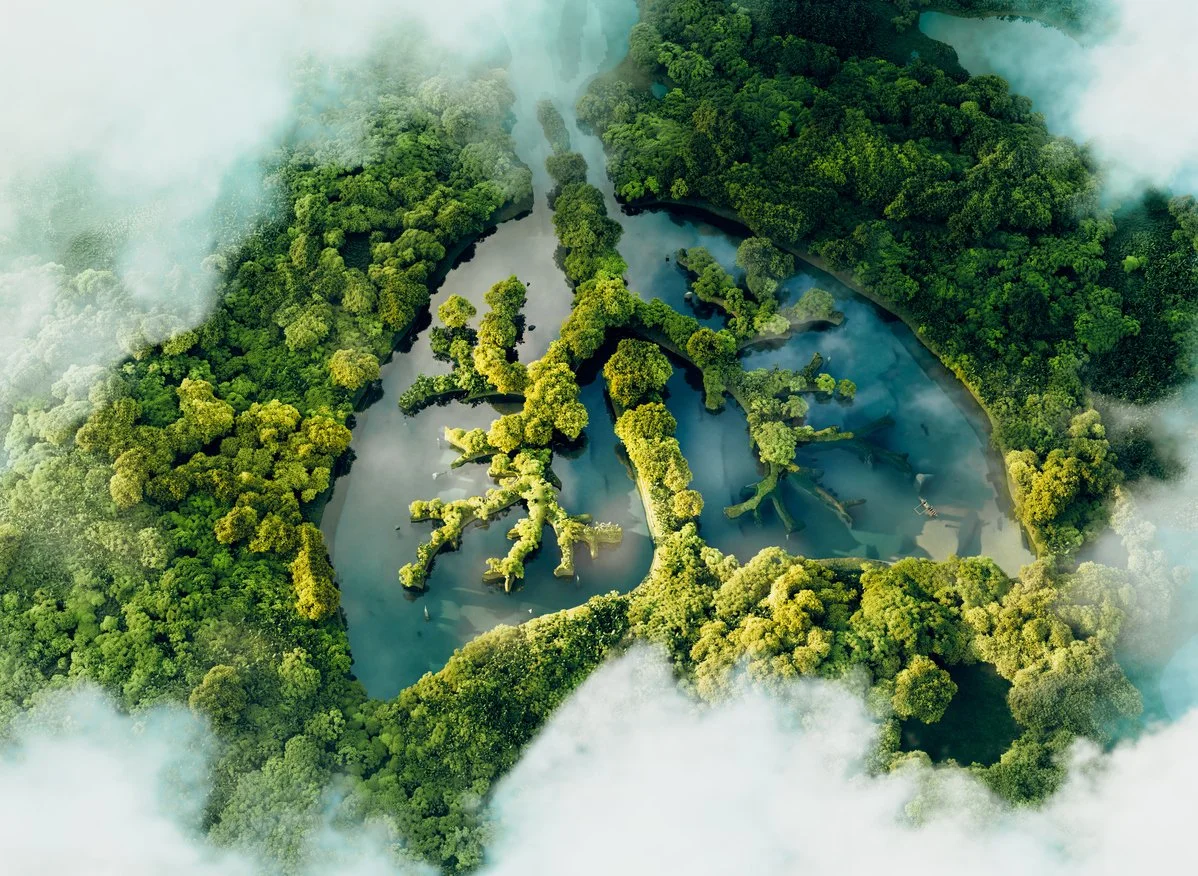
Lets get Scientific
Mild oxygen therapy
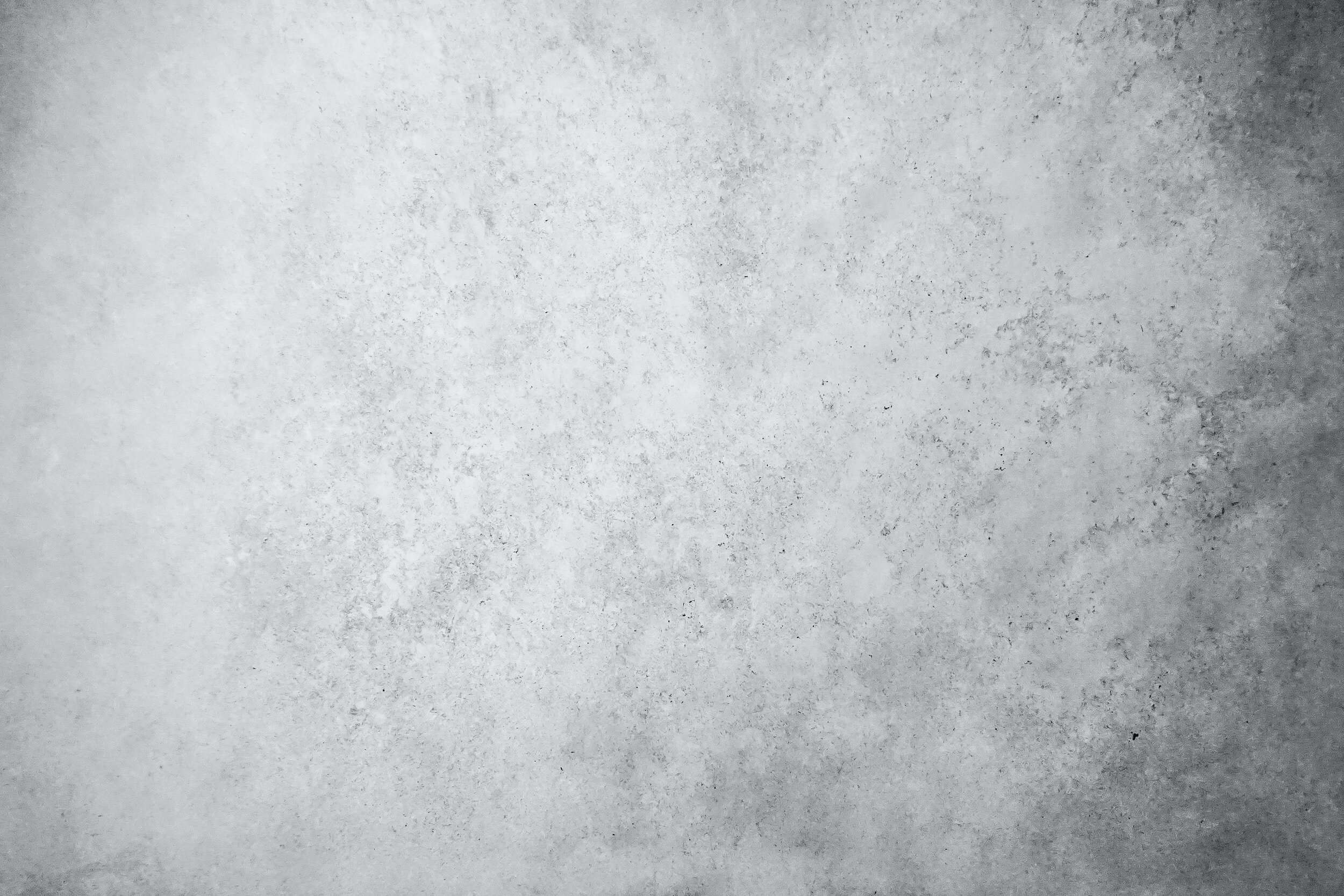
This section is for those of you who want to dive a little deeper and understand the mechanisms of action. “Let’s get scientific”, will explain, in simple terms, the body’s response to pressurised oxygen exposure with references that support the claims made of them.
Before explaining the physiology associated with exposure to oxygen at high pressures, it is important to understand that Mind over Matter Practice is primarily focused on training you to become more resilient to stress. The reason this is mentioned here first is because we do not claim to cure disease. Rather, we offer to move your mind beyond its current definition of discomfort. Through your willingness to expose yourself to the unknown, not only will you develop your self-assurance, but also the ability to control your brain’s biological regulation of the stress response internally (Meine et al. 2021).
Mild hyperbaric oxygen exposure is a controlled and carefully regulated process that involves treating your body to a slightly increased atmospheric pressure while breathing elevated levels of oxygen. This immersive experience harnesses the power of oxygen to promote enhanced oxygenation at the cellular level, aiding in various physiological processes (Ishihara 2019).
The mild hyperbaric oxygen experience delivered to you at Mind Over Matter differs from medical grade hyperbaric oxygen therapy (HBOT) utilised in hospital settings both in its design and objectives. Medical-grade HBOT is primarily used to treat various medical conditions, such as decompression sickness, gas embolism, non-healing wounds and various other diseases and, as such, it involves the administration of 100% pure oxygen ranging from 2.0 to 3.0 atmospheres of pressure or more.(Parr, Anderson & Veazie 2021)
During mild hyperbaric oxygen exposure in our unit, on the other hand, individuals enter a specially designed chamber where the atmospheric pressure is moderately increased, typically ranging from 1.3 to 1.5 times the normal atmospheric pressure at sea level. Within this pressurized environment, individuals will breathe in up to 97% oxygen, which replaces the ambient air they would normally inhale. The chamber is designed this way because it is not intended to treat disease by changing your internal environment. Instead, it is designed to stimulate and enhance your adaptive responses through hormesis, which is to naturally optimises the inner workings of your physiology by amplifying your inherent tendencies towards balance. In this way a new threshold is developed from repeated exposures.
Although skeptics often question its effectiveness by comparing it to medical-grade HBOT and hyperventilation, it is clear from the oxygen levels and pressures that mild hyperbaric oxygen delivery is superior in terms of safety and effectiveness as compared to HBOT and hyperventilation, respectively. To demonstrate this, let’s compare the oxygen delivery between our 97% oxygen at 1.5 atmospheres and the 100% oxygen at 2 atmospheres of the HBOT. To do this we will utilise the principles of partial pressure and gas laws. Let's delve into the calculations to understand the scientific and professional aspects of this comparison and examine it next to breathing ambient oxygen at sea level as well as hyperventilation at ambient barometric pressures.
First, let's calculate the partial pressure of oxygen in each scenario. The partial pressure of oxygen (PO2) can be calculated using the formula: PO2 = FiO2 × Patm, where (FiO2) is the fraction of inspired oxygen (0.21 at sea level) and (Patm) is the atmospheric pressure (760 mmHg at sea level). This 760mmHg is the total air pressure you are currently exposed to (Jones et al. 2021).
At baseline:
21% oxygen at 1.0 atmospheres: Partial pressure of oxygen = fraction of oxygen (0.21) × Pressure (1.0 atmospheres)
Partial pressure of oxygen = 0.21 x 1.0 x 760mmHg at sea level = 159.6mmHg
Under Mild Hyperbaric Conditions:
97% oxygen at 1.5 atmospheres: Partial pressure of oxygen = Fraction of oxygen (0.97) × Pressure (1.5 atmospheres)
Partial pressure of oxygen = 0.97 × 1.5 = 1105mmHg as a maximum amount delivered to trigger hormesis.
Under Medical-grade HBOT Conditions:
100% oxygen at 2 atmospheres: Partial pressure of oxygen = Fraction of oxygen (1.0) × Pressure (2 atmospheres)
Partial pressure of oxygen = 1.0 × 2 = 1520mmHg as a minimum amount delivered to treat disease
Hyperventilation at Ambient Sea Level Pressure in this scenario would reflect rapid breathing of the ambient air shown above which delivers 85% less PO2 than the mild hyperbaric chamber. It is important to note here that despite increasing the ventilation rate, hyperventilation of sea level air at normobaric conditions does not necessarily increase blood oxygen levels due to physiological mechanisms that limit its effectiveness. Let’s look at what these mechanisms are:
The primary reason why hyperventilation does not significantly increase blood oxygen levels is due to the regulation of carbon dioxide (CO2) in the body. When we hyperventilate, we breathe out more CO2 than our body is producing, leading to a decrease in the partial pressure of CO2 in the blood. This process is known as respiratory alkalosis (Tinawi 2021).
The decrease in CO2 levels triggers a response from the body known as the Haldane effect. The Haldane effect means that hemoglobin has a higher affinity for oxygen due to its abundance over CO2. As a result of oxygen binding more strongly to hemoglobin, it becomes less readily available for delivery to the tissues (Malte, Lykkeboe & Wang 2021).
Additionally, the respiratory alkalosis induced by hyperventilation can lead to vasoconstriction in certain areas of the body, further limiting oxygen delivery. This vasoconstriction occurs as a compensatory response to maintain the balance between oxygen and carbon dioxide (Godoy et al. 2017).
From the results of our equation and in consideration of our physiology, we can see that the minimum partial pressure delivered by the Medical-grade HBOT is 27% more than that of the mild hyperbaric chamber. However, as the Rennaisance physician Paracelsus put it, “nothing is without poison - the poison is in the dose”(Grandjean 2016). Therefore, it must be noted, accordingly, that the risk of complications such as barotrauma, uncontrolled seizures and oxygen toxicity typically increases at pressure differences beyond 2 atmospheres (Nisa et al. 2023). Nevertheless, mild hyperbaric oxygen clearly facilitates a much greater dissolution of oxygen into the bloodstream as compared to either breathing normally or hyperventilating in ambient air. Although it is short lived as a consequence of the hyperoxic-hypoxic paradox, it allows greater levels of oxygen to be delivered to tissues and organs. Heightening oxygen availability will amplify essential cellular processes to prepare cellular metabolism for greater demands(Bitterman 2009; Steurer et al. 1997).
The first of these cellular processes that is amplified by elevated oxygen exposure is the promotion of cellular respiration, a process by which cells generate energy in the form of ATP (Schottlender, Gottfried & Ashery 2021). The second is a mild elevation of reactive oxygen species (ROS) for cellular signalling. Providing oxygen is not increased to toxic levels, it is accepted throughout scientific literature that it aids cells to function optimally, enabling tissue repair and promoting natural healing processes (Zhou et al. 2023). Similarly, when increases in ROS are moderate, they are known to activate antioxidant defense systems, stimulating the production of endogenous antioxidants to counterbalance the oxidative stress and establish an enhanced threshold for homeostasis for future demands under stress (Fu et al. 2022; Pendyala et al. 2011).
Finally, we look to the activation of the hyperoxia-hypoxia paradox. This occurs during the intermittent periods of elevated oxygen availability within the mild hyperbaric chamber. While the overall oxygen concentration remains higher than normal atmospheric conditions, chemoreceptors are inactive. However, after approximately two minutes, the Bohr effect, along with elevated ROS, stimulates the central chemoreceptors in the brainstem to initiate CO2 as its transport is hindered (Hadanny & Efrati 2020; Marczak & Pokorski 2003). This is because as free CO2 levels elevate within the blood under elevated oxygen pressures, it cannot weaken the affinity of O2 for hemoglobin due to its being forced into the plasma under pressure.
As the O2 and pressure return to normal sea level readings at the end of the session, your cells will interpret the environment as hypoxic and activate transcription factors necessary for survival in hypoxic conditions. This response prompts the body to undergo various adaptive mechanisms to cope with the temporary decrease in oxygen availability (Behrendt et al. 2022; Forkner et al. 2007).
A cascade of additional protective mechanisms, such as antioxidant defenses, stimulation of angiogenesis (formation of new blood vessels) and increased red blood cell production take place (Balestra et al. 2006). These responses help to improve cellular resilience, optimize energy metabolism, and enhance the tissue repair processes (Hadanny & Efrati 2020).
It is worth noting that although hyperventilation has minimal effects on oxygen levels in the blood, the elimination of CO2 which occurs in the chamber due to the hyperoxia-hypoxia paradox effectively conditioning the cells for higher demands as ventilation remains elevated well after the session’s completion (Brugniaux et al. 2018).
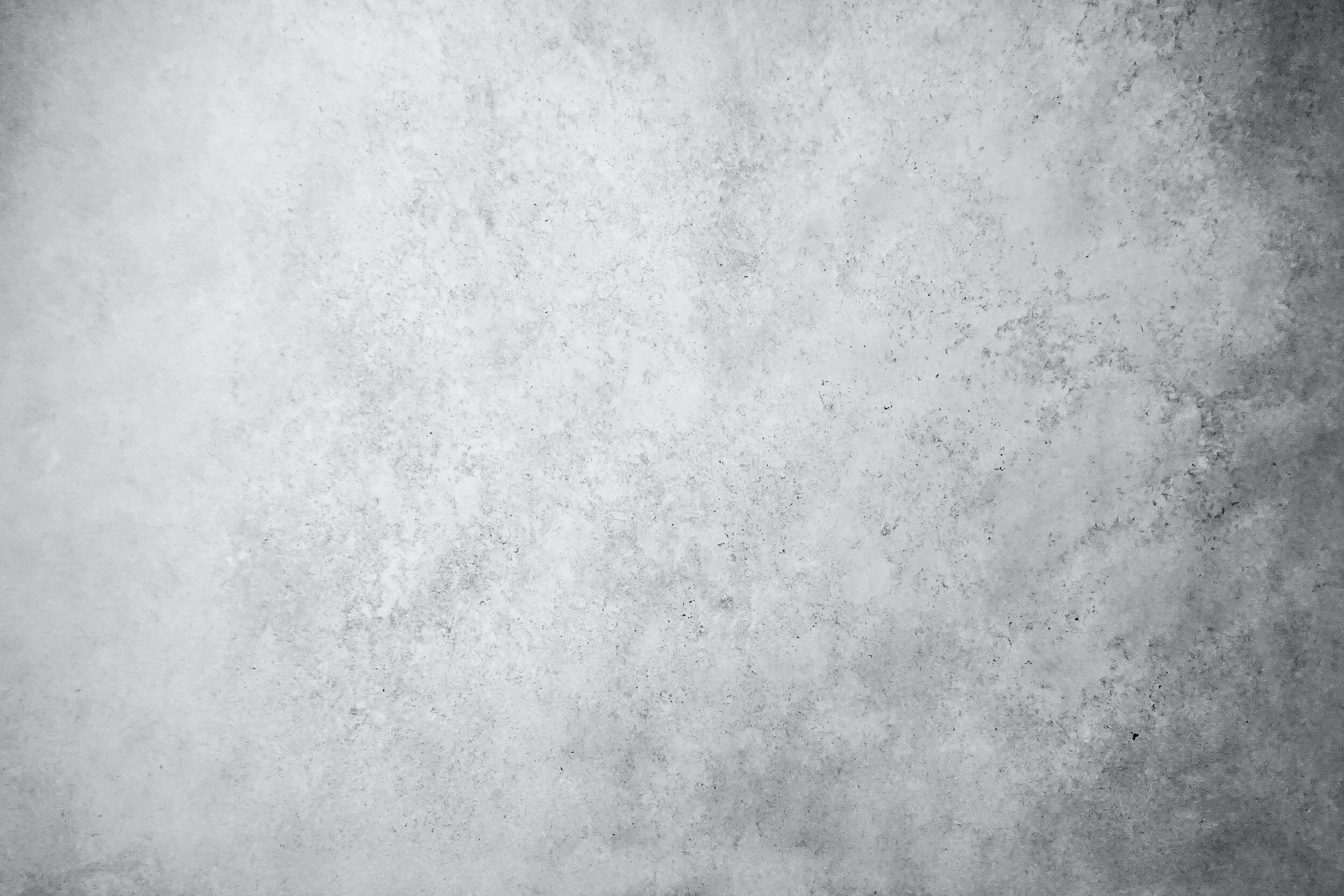